Research
Our group focuses on the mesoscopic investigation of both quantum electron transport and mechanical resonators. We explore phenomena based on electron correlation, quantum effects, and topology in novel condensed matter systems such as twisted bilayer graphene stacks and one- and two-dimensional single crystals. We also use such systems to produce electromechanical resonators endowed with enhanced quantum fluctuations and ultra-long life times, which are at the heart of modern advances in nanomechanics.
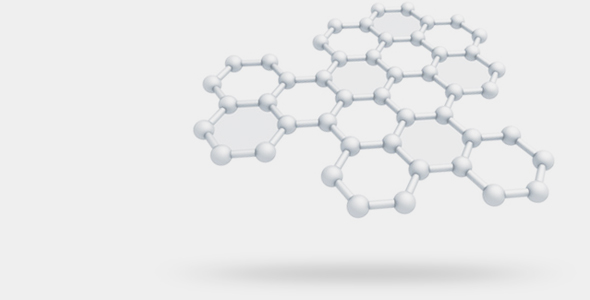

An electronic
quantum simulator
Our aim is to construct a new experimental tool based on a graphene superlattice to not only measuring macroscopic observables, such as electrical resistivity and magnetization, but also probing the many-body quantum wavefunction of correlated electronic states. This new quantum simulator platform may unravel the microscopic nature of high-Tc superconductivity and other correlated electron states, such as heavy fermions in a Kondo lattice, integer and fractional Chern insulators, and orbital ferromagnets.
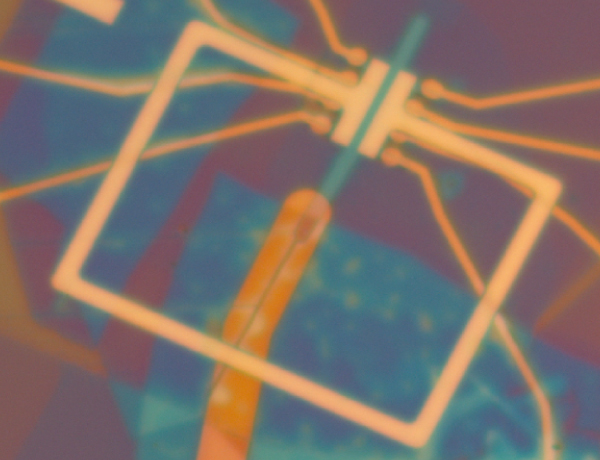
Quantum electrodynamics
in two dimensions (QED)
We aim to manipulate correlated electron states in two dimensions with virtual photons in the terahertz range. We will produce van der Waals heterostructures, where conducting electrons in a magic-angle twisted bilayer graphene or Bernal bilayer graphene are coupled to the quantum fluctuations of a photonic cavity. We are harnessing correlated using the photons of a microfabricated split-ring electromagnetic cavity. Such LC-like cavities feature strongly enhanced quantum fluctuations compared to free space due to the deep subwavelength spatial confinement of the fundamental mode. The experiment will be carried out with the cavity in the ground state without illuminating it.
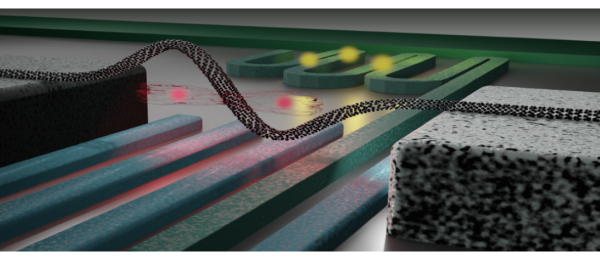
Towards a
mechanical quantum bit
This project focuses on the realization of a completely new qubit, where the information is stored in the electromechanical vibrations. We propose to realize the mechanical qubit by coupling the mechanical vibrations of a nanotube resonator to a double-quantum dot formed along the suspended nanotube. Nanotubes are the ideal system for such an experiment, since their high mechanical quantum fluctuation amplitude boosts the single-phonon electromechanical coupling strength. We may reach sub-kHz decoherence rate for the mechanical qubit. Such a demonstration would be a breakthrough in quantum science, offering new perspectives for quantum networks and quantum sensing.